How do Batteries Work?
09-10-23
Broadly speaking, a battery is anything that can store energy and then release that energy in a controlled manner. Our bodies can be considered batteries in the way they store chemical energy in the form of fat and other substances. Springs in mechanical systems are like batteries that store and release mechanical energy. Usually however, when we say battery, we are referring to electrochemical batteries, batteries that store electric energy as chemical potential energy. These batteries are also referred to as electrochemical cells. These batteries power nearly every electronic device that is not always connected to the electrical grid. From flashlights to tablets to cars, all these things need electrochemical batteries to function.
So how does a battery turn chemical energy into electrical energy and vice versa? Well at first, batteries could only discharge and unlike your phone battery could not be recharged, so lets look at how the first electrochemical cells functioned.
The Voltaic Pile
The first electrochemical cell was created by Italian physicist Alessandro Volta which is now known as the voltaic pile. The voltaic pile was a stack of individual cells, the smallest functioning unit of a battery, each of which consisted of a zinc and copper plate separated by a salt solution-soaked cloth. Connected to the top and bottom of the stack is the wire that carries the electric current.
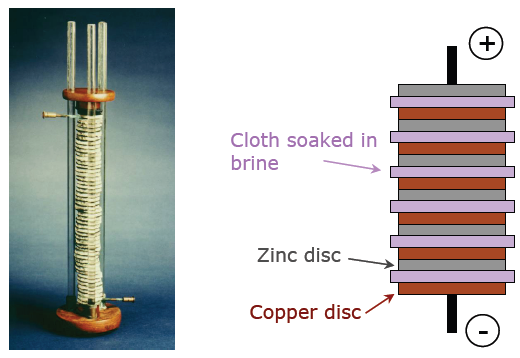
In each individual cell can be seen the three fundamental components in every battery: The anode, the cathode and the electrolyte. In this pile, copper is acting as the anode and zinc is acting as the cathode while the saltwater in the paper is the electrolyte. Electricity can be understood as the flow of electrons through conductive materials like wires. The flow of electrons is caused by a difference in electric potential energy. Copper is more electronegative than zinc which means it attracts electrons to itself more strongly. The resulting potential difference causes zinc to undergo a spontaneous half-reaction known as reduction. A reduction reaction is a reaction where electrons are removed from a chemical species.
This is known as a half reaction because another reaction is usually required to consume the electrons since electrons are more stable when they exist in atoms. A reaction that adds electrons to a chemical species is called an oxidation reaction. The oxidation and reduction reaction occur at the cathode and anode respectively, with the reduction reaction producing electrons and flow from the anode and through the circuit to be consumed in the oxidation reaction occurring at the cathode. The oxidation half reaction occurring at the cathode in the voltaic pile is shown below.
In this reaction, the excess electrons on the copper plate attack water to turn it into hydroxide and hydrogen gas. The element being oxidized in this oxidation reaction is oxygen since it gains an electron. These two half reactions can be added together to form one complete reaction.
This complete reaction is known as a redox(reduction + oxidation) reaction. This reaction continues as long as there is zinc to be dissolved and a path allowing the flow of electrons. Note that this reaction does not include copper but the copper is necessary as the difference in electronegativity between copper and zinc acts as a driving force in this reaction. After this example, another will be shown of an electrolytic cell where copper is involved in the reaction.
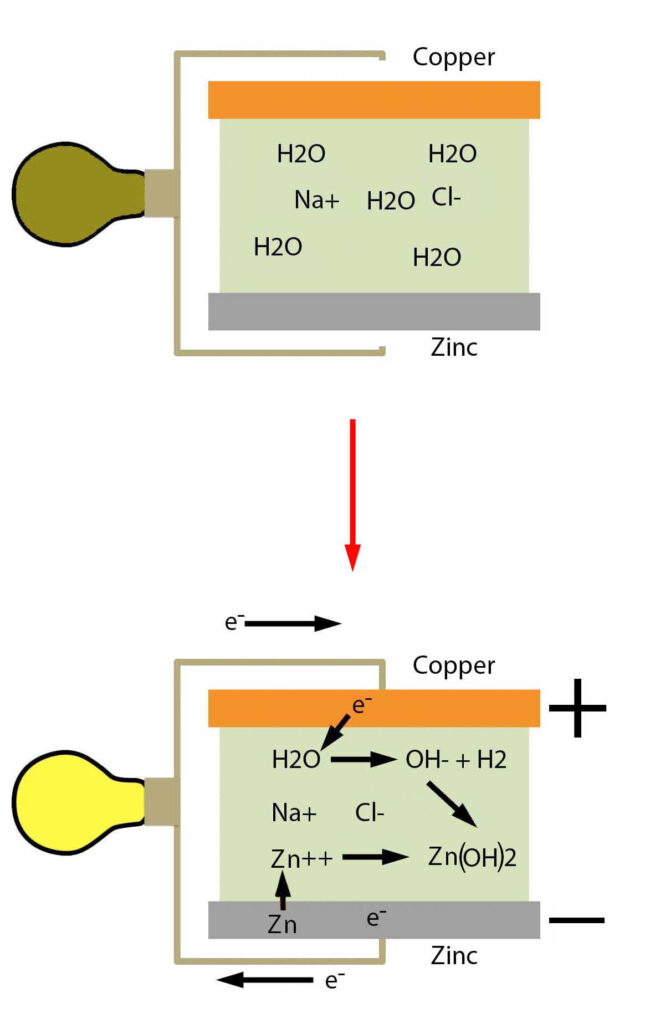
The Galvanic Cell
In high school and college chemistry classes, one often required lab is the construction of a galvanic cell, similar to what is shown below.
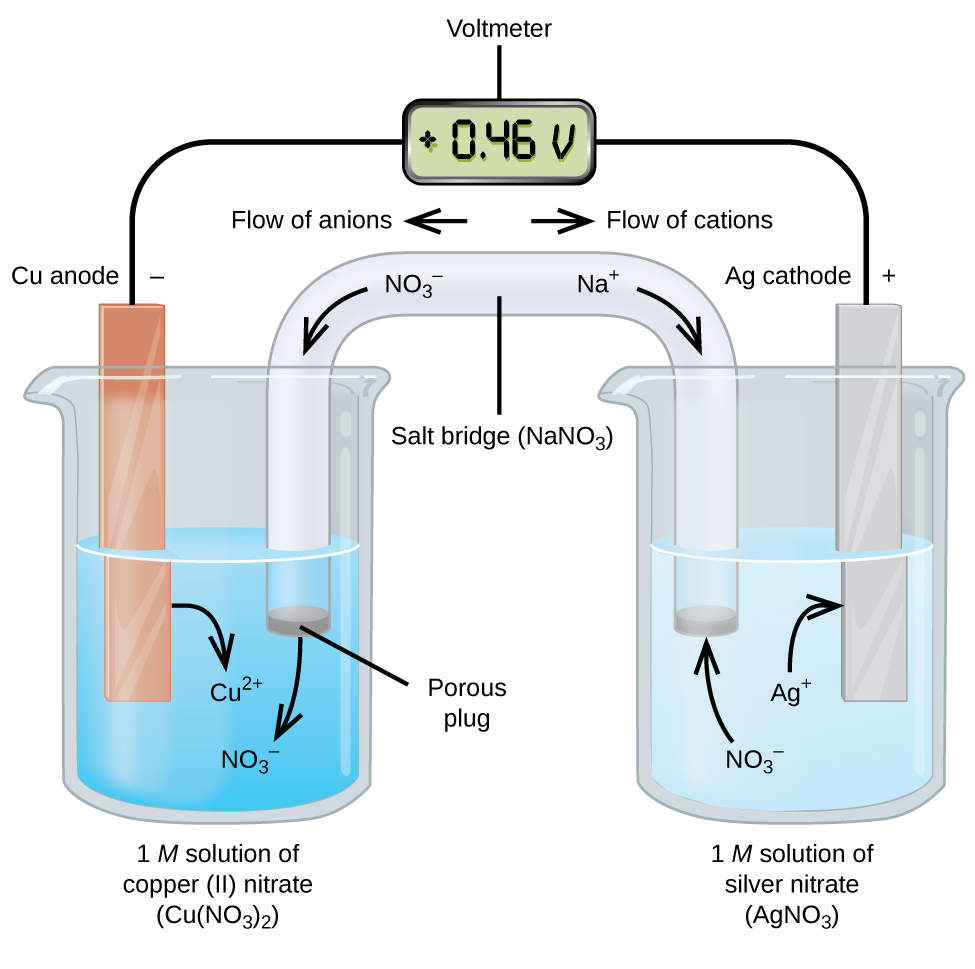
In this cell we can see that copper, unlike in the voltaic pile example, is acting as the anode while silver is acting as the cathode. Also both electrodes exist in their own solution containing their respective ions to act as the electrolyte. The electrolyte in this cell will be participating in the redox reaction. Each half reaction and the full reaction are below.
We can see that, copper is the anode in this cell because it is being oxidized(gaining electrons) in the reaction and vice versa for silver.
Because the electrolytes are now directly involved in the chemical reaction, they must be separated into half cells so that the reaction between Cu and Ag+ doesn’t occur at the surface of the copper electrode. It is important that this doesn’t occur because then the electron transfer would be direct and not force the electrons to travel around the wire.
Since there are now two solutions unlike in the voltaic pile, a salt bridge is necessary to allow the balance of charge. This salt bridge allows spectator ions(ions not involved in any reaction) to travel between the solutions to balance the charge. In this case the spectator ions are Na+ and No3-.
To summarize, batteries produce electrical power by separating the reactants of a redox reaction such that it can only occur if the electron transfer needed can only occur by traveling through an electric circuit. Which chemicals used and how they are separated vary across the different battery types, each setup carrying with it different pros and cons.
Sources/Further Reading